A traffic cop for the cell surface
Researchers illuminate a basic biological process
On the surfaces of our trillions of cells is a complex crowd of molecules moving around, talking to each other, occasionally segregating themselves, and triggering basic functions ranging from pain sensation to insulin release.
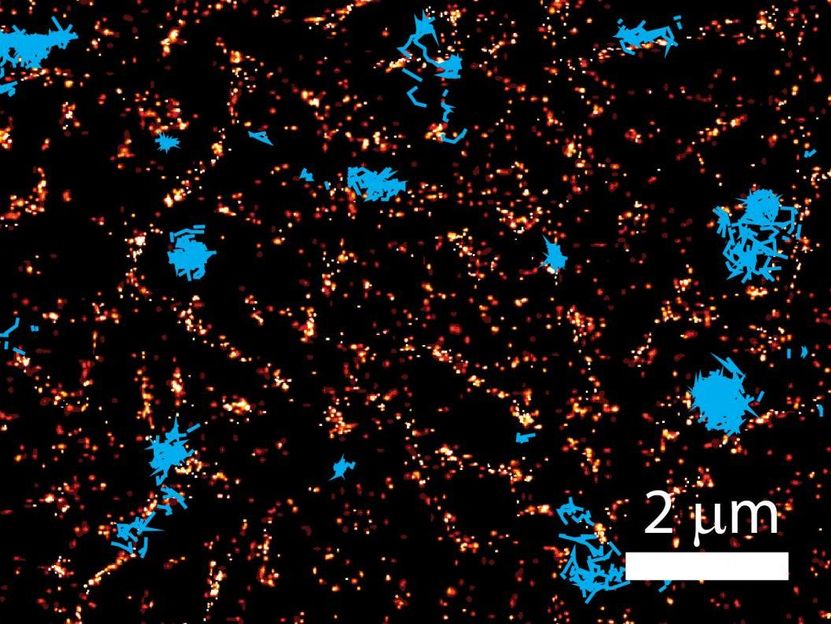
This image shows a superresolution image of actin (red), interacting with cell membrane proteins. The blue traces are trajectories of proteins colliding with the actin network.
Krapf Lab/Colorado State University
The structures that organize this microscopic traffic jam are no longer invisible, thanks to Colorado State University researchers. A multidisciplinary team of single-molecule biophysicists and biochemists have shed light on a long-obscured cellular process: a mammalian cell membrane's relationship with a scaffolding underneath it, the cortical actin cytoskeleton. For the first time, the CSU team has made real-time observations of this cytoskeleton acting as a barrier that organizes proteins on the cell's surface, effectively playing traffic cop on the cell's membrane activities.
The breakthrough visualization and analysis of this most fundamental biological process - how a cell membrane interacts with its intracellular environment and controls cellular functions - was jointly achieved by the labs of Diego Krapf, associate professor of electrical and computer engineering and biomedical engineering, and Michael Tamkun, professor of biomedical sciences in the College of Veterinary Medicine and Biomedical Sciences, and of biochemistry, in the College of Natural Sciences. The researchers' study will appear in a forthcoming edition of Physical Review X, with first author Sanaz Sadegh, a Ph.D. student in Krapf's lab.
In their study, the researchers used a powerful superresolution imaging technology called photoactivated localization microscopy (PALM), which, by circumventing the natural diffraction limit of light, allows scientists to take crisp pictures and videos of biological processes at the nanoscale. Superresolution microscopy was the subject of the 2014 Nobel Prize in Chemistry.
The CSU researchers focused on the movements of potassium ion channels, a type of protein critical to cellular functions on the cell surface, and how these ion channels interact with the cortical actin cytoskeleton. The cytoskeleton is a spider-web-like network of filaments just under the cell membrane that gives the cell some of its shape and structure. Scientists had previously hypothesized that the cytoskeleton plays a critical role in helping the membrane proteins that stud the cell surface organize themselves and transmit signals to keep the cell healthy and functioning. But visually capturing this actin-protein interaction in live cells had been impossible.
"Proteins on the cell surface, like ion channels, have a lot of mass that hangs down into the cell," Tamkun explained. "It's that intracellular mass that collides with the actin network."
Using a custom-designed superresolution microscope, the researchers made movies that captured the exact moments when the ion channels collided with the actin network. What's more, they performed statistical analysis on these movements to provide evidence of the actin's key structural elements. The cortical actin network in a cell is a fractal, which means it is structurally similar at varying length scales.
"The fractal nature of the actin network explains our measurements," Sadegh said. "It leads us to question why we see so many fractals in nature. Is it an efficient way to organize functions? It's an interesting question for future studies."
The CSU researchers' analysis showed that the cell membrane proteins' random movements exhibit sophisticated patterns. Among their observations was that the proteins tended to bounce back into the places they had previously visited. For the first time, the CSU researchers offered statistical and visual evidence that this bounce-back is directly caused by the actin's fractal nature.
The chief technical challenge was achieving high-resolution images in very short time bursts, according to Krapf. "If we wait 10 seconds, the cell cytoskeleton changes, so we need to image it fast. We were employing two-second intervals, and within those seconds we needed to obtain a spatial resolution high enough to see collisions between individual membrane proteins and the actin structure."
The researchers want to understand everything about the cell membrane, because that's how the cell communicates with its outside environment, and it may hold the key to disease progression and other aspects of human health. "It's important for us to understand how the cell organizes its membrane to keep things in the places they need to be," Sadegh said. She suggested that future studies could focus on specific sites on the membrane - for example, where endocytosis takes place - and how the actin network regulates localized activity.
Other news from the department science
These products might interest you
Most read news
More news from our other portals
See the theme worlds for related content
Topic world Fluorescence microscopy
Fluorescence microscopy has revolutionized life sciences, biotechnology and pharmaceuticals. With its ability to visualize specific molecules and structures in cells and tissues through fluorescent markers, it offers unique insights at the molecular and cellular level. With its high sensitivity and resolution, fluorescence microscopy facilitates the understanding of complex biological processes and drives innovation in therapy and diagnostics.
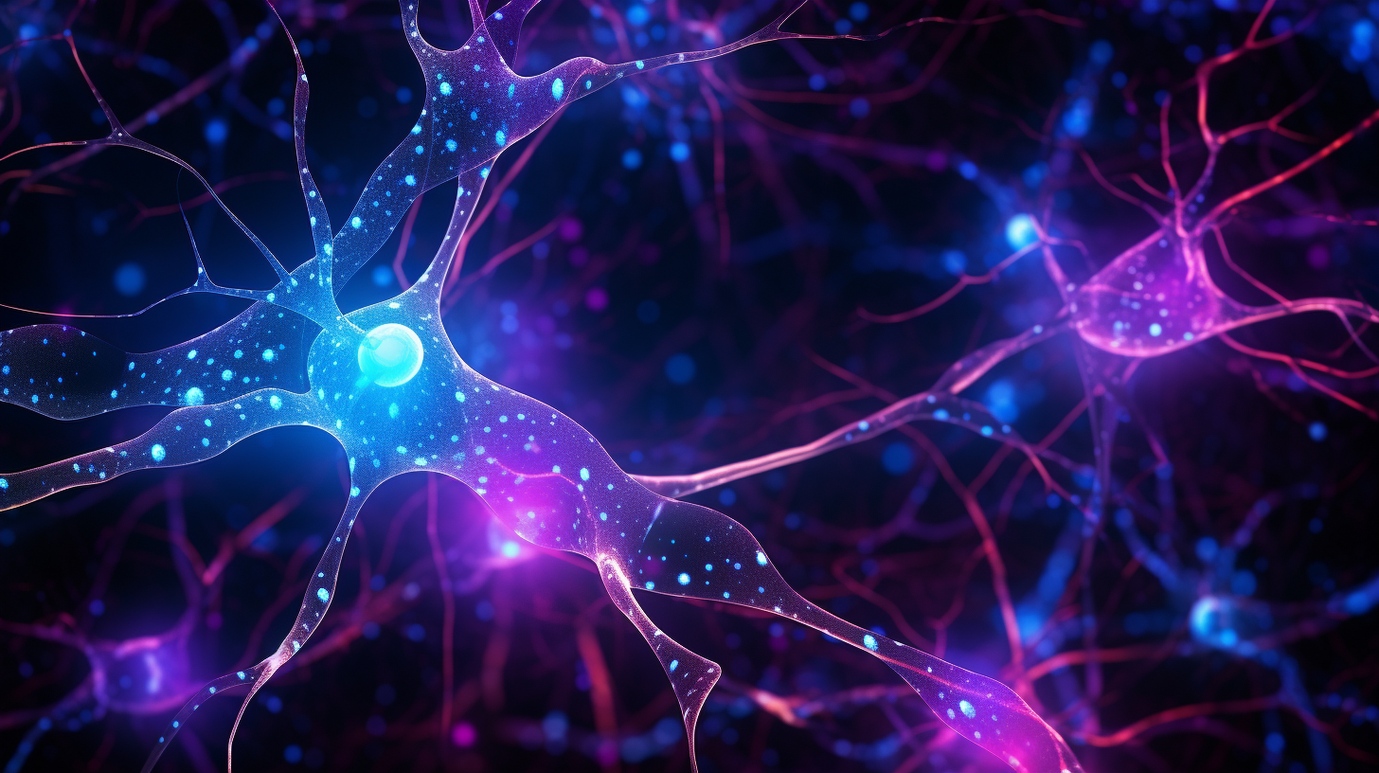
Topic world Fluorescence microscopy
Fluorescence microscopy has revolutionized life sciences, biotechnology and pharmaceuticals. With its ability to visualize specific molecules and structures in cells and tissues through fluorescent markers, it offers unique insights at the molecular and cellular level. With its high sensitivity and resolution, fluorescence microscopy facilitates the understanding of complex biological processes and drives innovation in therapy and diagnostics.