Researchers show how gene activation protein works
Structure sought for four decades; foundation for understanding gene activation
Rutgers University scientists have discovered the three-dimensional structure of a gene-specific transcription activation complex, providing the first structural and mechanistic description of the process cells use to turn on, or activate, specific genes in response to changes in cell type, developmental state and environment.
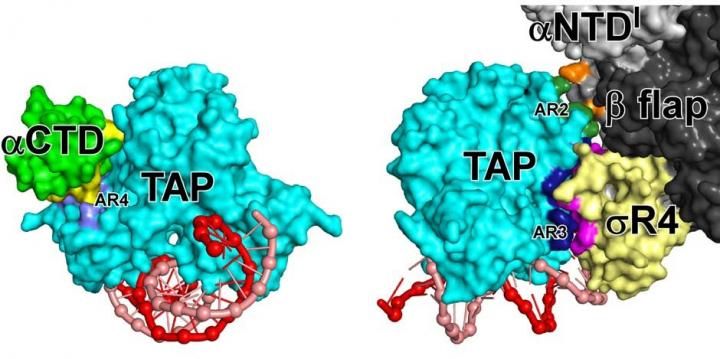
Left: Interactions made by a transcription activator protein (TAP; light blue) with RNA polymerase α subunit C-terminal domain (αCTD; green). The interactions involve an exposed surface of TAP (AR4; violet) and an exposed surface of αCTD (yellow), occur before or during the binding of RNA polymerase to DNA, and help RNA polymerase bind to DNA. Right: Interactions made by TAP with the RNA polymerase β subunit (black and gray) and a domain of the transcription initiation factor σ that makes sequence-specific interactions with DNA (σR4; yellow). The interactions involve exposed surfaces of TAP (AR2 and AR4; green and blue) and exposed surfaces of β and σR4 (orange and pink), occur after the binding of RNA polymerase to DNA, and help RNA polymerase and σ unwind DNA.
Yu Feng and Richard H. Ebright
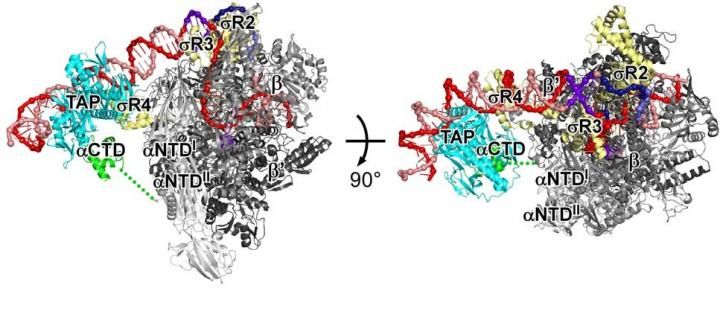
A crystal structure of a gene-specific transcription activation complex reveals the interactions between RNA polymerase (black, gray, and green), sigma (yellow), and DNA (red and pink; sequence elements in violet and blue) that mediate DNA binding and DNA unwinding in transcription initiation and reveals the interactions between the transcription activator protein (TAP; light blue), RNA polymerase, and sigma that mediate transcription activation.
Yu Feng and Richard H. Ebright
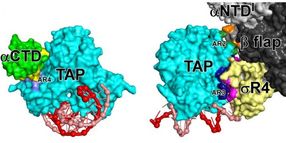
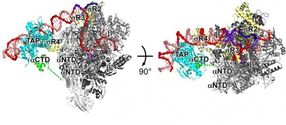
Transcription is the first in a series of steps cells take to read out genetic information in DNA.
Richard H. Ebright and other Rutgers scientists show how a transcription activator protein interacts with the enzyme -- RNA polymerase -- that cells use to perform transcription. They also show how the transcription activator protein helps RNA polymerase bind to the DNA helix at a specific site preceding a gene, and how the transcription activator protein helps RNA polymerase unwind the DNA helix to initiate transcription of the gene.
The Rutgers scientists show that the transcription activator protein functions by binding to a specific DNA sequence preceding the target gene and making adhesive, Velcro-like interactions with RNA polymerase that stabilize contacts by RNA polymerase with adjacent DNA sequences.
The Rutgers scientists further show that the transcription activator protein makes two separate, successive sets of adhesive, Velcro-like interactions with RNA polymerase: one that helps RNA polymerase bind to the DNA helix, and a second set that helps RNA polymerase unwind the DNA helix.
"Determining the structure of a gene-specific transcription activation complex has been a goal of researchers for almost four decades," said Ebright, Board of Governors professor of chemistry and chemical biology and laboratory director at the Waksman Institute of Microbiology at Rutgers.
The research team also included Rutgers research associate Yu Feng and Rutgers research assistant professor Yu Zhang.
"This is a landmark paper," said Steve Busby, professor of biochemistry at the University of Birmingham, United Kingdom, who was not part of the study. "For the first time, we have a full molecular picture of transcription activation at a target promoter. Previous reports have described components of the process, or have relied on reconstructions from lower resolution data. Here, Ebright and colleagues have cracked the problem by using components from bacteria that grow at high temperature. This results in solutions to some basic questions about how activators work, but also the use of the thermophile system uncovers some unexpected variation, illustrating how a fundamental mechanism can be elaborated in different branches of the evolutionary tree."
The structure determined by the Rutgers researchers is the structure of a transcription activation complex containing the TTHB099 activator protein (TAP) from the bacterium Thermus thermophilus. Because TAP is closely related in sequence and structure to the prototypical, best-known bacterial transcription activator protein -- the catabolite activator protein (CAP), also known as the cyclic AMP receptor protein (CRP) -- the results provide a framework for understanding bacterial transcription activation. Because the transcription machineries in bacteria and higher organisms are structurally and mechanistically related, the structure also provides a framework for understanding transcription and transcriptional regulation in higher organisms, including humans.
The structure defines the interactions that RNA polymerase and the transcription initiation factor sigma make with DNA upstream of a gene. The structure also defines the interactions that TAP makes with DNA, RNA polymerase and sigma.
TAP recognizes and binds to a specific DNA sequence upstream of the gene. It recognizes the specific DNA sequence by inserting a pair of α-helices into grooves of the DNA helix and sensing functional groups on DNA base-pair edges.
The structure shows that an exposed surface of DNA-bound TAP, called AR4, makes protein to protein contacts with the RNA polymerase α subunit C-terminal domain. This interaction is made before or during the initial binding of RNA polymerase to DNA and helps RNA polymerase bind to DNA. The structure shows that two other exposed surfaces of DNA-bound TAP, called AR2 and AR3, make protein to protein contacts with the RNA polymerase β subunit and with sigma. These two interactions are made only after the initial binding of RNA polymerase to DNA and help RNA polymerase and sigma unwind DNA.
Both the AR4 interaction and AR2 and AR3 interactions are simple, adhesive, Velcro-like interactions that function by stabilizing contacts by RNA polymerase and sigma with the DNA segment adjacent to the DNA segment bound by TAP. The different functional consequences of the two sets of interactions -the AR4 interaction facilitates DNA binding and the AR2 and AR3 interactions facilitate DNA unwinding -- arise from differences in the timing of interactions, not from differences in the character of interactions.
"The paper provides a dazzling snapshot of the process of transcription activation -- the process whereby a gene is turned on in response to an instructive signal from the environment," said Ann Hochschild, professor of microbiology and immunobiology at Harvard Medical School, who also was not part of the study. "Ebright and colleagues present a crystal structure that captures a network of interactions between an activator protein bound to DNA and RNA polymerase (the primary enzyme of gene expression) as it is poised to begin gene transcription. Validated by precision genetic analysis, the structural findings support an elegant and unified mechanism for activator function."