Mechanics of the cell
Investigation of simple model cells resolves deformation mechanisms
Living cells must alter their external form actively, otherwise functions like cell division would not be possible. At the Technical University of Munich (TUM) the biophysicist Professor Andreas Bausch and his team have developed a synthetic cell model to investigate the fundamental principles of the underlying cellular mechanics.
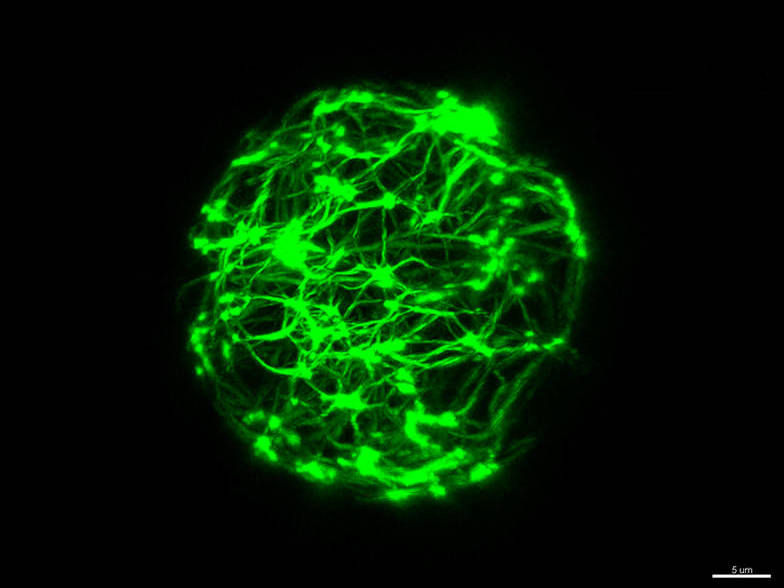
Reconstitution of active cytoskeletal vesicles. The active cytoskeleton (green)exerts forces to the surrounding lipid membrane.
Etienne Loiseau / TUM
Living cells do not lead a relaxed life: They are perpetually busy adapting their physical shape. When they divide, cells must massively restructure their overall form. When they absorb material, their outer membrane must deform strongly. And when they move about, they must first decide what is front and what is back before their built-in molecular motors kick in, setting the cell into motion.
Cells are living organisms with all kinds of dynamic functions. The ability of a cell to morph its shape is decisive. During cellular division these processes play an important role, whether in wound healing, embryonic development or spreading of cancer cells throughout the body.
The ways in which cells spontaneously alter their shapes using only a few components is the subject of detailed investigation by the research team of the biophysicist Andreas Bausch, Chair of Cellular Biophysics at TU Munich and a member of the Cluster of Excellence Nanosystems Initiative Munich. The researchers hope the reconstruction will help them better understand the functions of complex cellular systems.
Model cells for investigations of cellular mechanics
The outer shell of the model cell comprises a double-layered lipid membrane analogous to natural cell membranes. Inside the researchers placed biomolecules that perform important functions in animal cells. In their experiments on the deformation of cells they used oblong actin filaments, which are normally found in the cytoskeleton.
In addition, they added the protein anilin, which facilitates the joining of actin filaments to impart the cell structural stability, and myosin as a molecular motor, which the cells need to generate the energy required for cellular deformation.
In their experiment, the researchers analyzed under which conditions the model cells spontaneously undergo deformations in which the cytoskeleton membrane either takes on a concave form or, in certain regions, forms a bubble-like protrusion in a process referred to as "blebbing". The forces exerted on the external form of the cells counteract respective forces in the lipid membrane.
"The interplay between cytoskeleton and cell membrane holds the key to all changes in form," says Etienne Loiseau of Bausch's working group and lead author of the current study. "To date cytoskeletons and vesicles were normally observed separately. The interaction of these two essential components was hardly investigated."
Focusing on the essentials
The model cell created in the project funded by the Cluster of Excellence Nanosystems Initiative Munich works with a small number of components. The respective concentrations of the involved proteins can be adjusted as required and precisely controlled.
Bausch and his colleagues demonstrated that the interactions between the proteins in the presence of all other components are the key. It is only through the interplay of the various components that biological functions emerge. Evidently, the concentration of components is decisive for the manner in which cells alter their form.
"Amazingly, the same system of proteins that affect the protuberance of membranes (blebbing) lead, in slightly different concentrations, merely to extreme deformations," says Bausch. "Understanding the interactions of the proteins in context is essential - it is the reciprocal actions of the proteins that define the functions."
Understanding the mechanisms of action
Although biologists managed to identify the involved proteins and genes in elaborate cell-biological and biochemical experiments, due to the complexity of the cells it is generally not possible to also understand the fundamental mechanisms of action. "Our bottom-up approach based on the synthetic cell model is useful for understanding and explaining important functional relationships," says Professor Bausch.
As yet, the experiments on cellular deformation only work in static systems. In their next step the scientists hope to reconstruct the dynamic processes, as well. They want to allow the formation and dispersion of bubbles in the cytoskeleton membrane as it occurs in nature, thereby creating artificial model cells that can exist and move about autonomously.