It takes two to tango: Pairs of entwined proteins handle the stress
Imagine two tango dancers sweeping across the dance floor and suddenly encountering a slick spot. To avoid a slip or even a nasty tumble, the pair must work together to support one another and glide safely through the stressful moment.
In a similar way, a certain type of helper protein called a stress-specific molecular chaperone prevents its client proteins from collapsing during stressful situations by uncoiling a long, supportive arm that wraps around them, a University of Michigan-led research team has shown,
When the stress has passed, the chaperone refolds the arm, allowing both proteins to regroup and return to normal life inside the cell.
"The new study illustrates how flexibility helps proteins – which are crucial to most tasks required for cells to exist – perform well under stressful conditions," said U-M molecular biologist Ursula Jakob, senior author of a paper on the topic scheduled for online publication March 1 in the journal Cell.
A protein's function depends on its shape, and a protein's shape depends on how it folds into complex three-dimensional configurations. Molecular chaperones are best known for assisting the protein-folding process.
Most molecular chaperones are active only when they are tightly folded. But University of Michigan scientists recently discovered that certain stress-specific proteins become active as chaperones only when they partially unfold and lose some of their structure. That finding turned common assumptions about molecular chaperones on their head.
In the Cell paper, a team led by Jakob describes how one of these stress-specific helper proteins, Hsp33, works.
Hsp33 is a bacterial protein that partially unfolds and becomes active when exposed to oxidants like bleach. Hsp33 helps other proteins survive bleach-induced protein unfolding, and in doing so makes the bacteria resistant to this powerful oxidant.
Hsp33 chaperones prevent other unfolding proteins from forming large, destructive clumps of inactive protein that can kill the bacterium. In concert with other chaperones, Hsp33 then helps its client proteins regain their structure once the stress has passed.
"The way these proteins turn on makes sense, as they can very rapidly respond to conditions that unfold other proteins," said Jakob, a professor in the Department of Molecular, Cellular and Developmental Biology in the College of Literature, Science, and the Arts, and an associate professor in the Department of Biological Chemistry at the Medical School. "But it raises several mystifying questions: How can proteins work while they are partially unfolded, and being disordered, how can they possibly bring order to other proteins?"
The U-M researchers discovered that Hsp33 cleverly uses its own partially unfolded regions to tightly bind other unfolding proteins, protecting them. In the dancing analogy, Hsp33 is the protein that reaches out a long, flexible arm that wraps around its client protein.
Hsp33's arm helps stabilize both protein "dancers" as they move through the stressful event, preventing the client protein from collapsing on the dance floor.
"And of course, like dance partners, there is tight structural interplay between Hsp33 and its client proteins," said Dana Reichmann, a research fellow in Jakob's lab and lead author of the Cell paper. "Once the stressful performance is over, Hsp33's arm refolds, allowing both dancers to recover their postures and resume their own performances."
Most read news
Other news from the department science
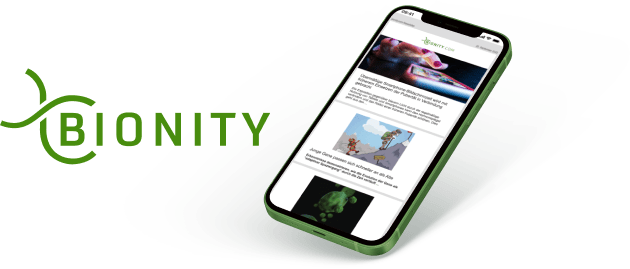
Get the life science industry in your inbox
By submitting this form you agree that LUMITOS AG will send you the newsletter(s) selected above by email. Your data will not be passed on to third parties. Your data will be stored and processed in accordance with our data protection regulations. LUMITOS may contact you by email for the purpose of advertising or market and opinion surveys. You can revoke your consent at any time without giving reasons to LUMITOS AG, Ernst-Augustin-Str. 2, 12489 Berlin, Germany or by e-mail at revoke@lumitos.com with effect for the future. In addition, each email contains a link to unsubscribe from the corresponding newsletter.