(How) Cells Talk to Each Other
ISTA Scientists Successfully Model Cell Dynamics
Like us, cells communicate. Well, in their own special way. Using waves as their common language, cells tell one another where and when to move. They talk, they share information, and they work together – much like the interdisciplinary team of researchers from the Institute of Science and Technology Austria (ISTA) and the National University of Singapore (NUS). They conducted research on how cells communicate – and how that matters to future projects, e.g. application to wound healing.
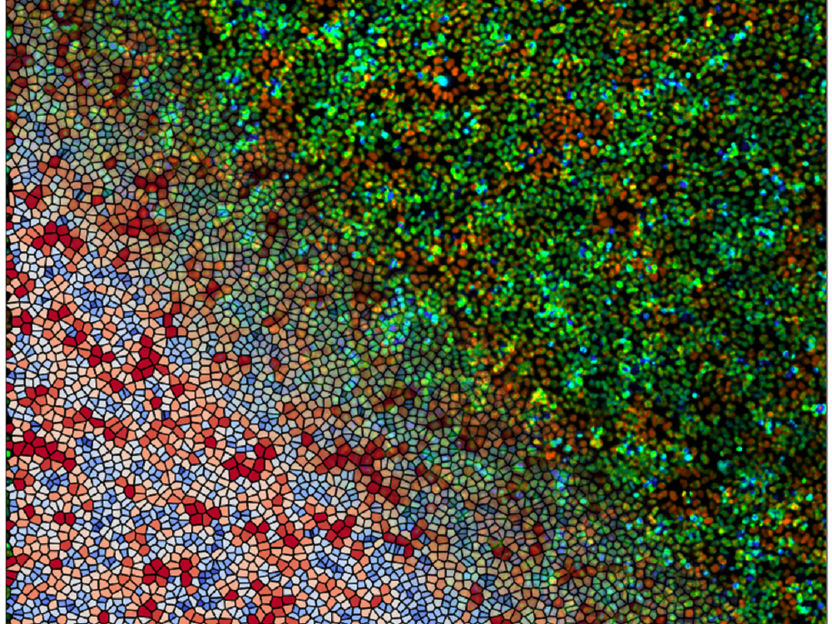
A magnificent flurry of colors. It shows the activation of a chemical signaling pathway (ERK pathway; top-right) merged with a simulation of 2D cell areas (bottom-left) in a monolayer of cells.
© Hannezo Group | ISTA
What comes to your mind when you think of biology? Animals, plants, theoretical computer models? The last one, you might not associate with it right away, although it is a major part of biological research. It is precisely these calculations that help to understand complex biological phenomena, down to the most concealed details. Edouard Hannezo, Professor at the Institute of Science and Technology Austria (ISTA), applies them to understand physical principles in biological systems. His group’s latest work gives novel insights into how cells are moving and communicating inside living tissue.
During his PhD at ISTA, Daniel Boocock, along with Hannezo and long-term collaborator Tsuyoshi Hirashima from the National University of Singapore, developed a detailed new theoretical model, which is published in the journal PRX Life. It allows a better understanding of long-range cell-cell communication and describes both the complex mechanical forces the cells apply to each other and their biochemical activity.
Cells communicate in waves
“Let’s say you have a Petri dish that is covered with cells—a monolayer. They appear to just sit there. But the truth is they move, they swirl, and they spontaneously make chaotic behaviors,” Hannezo explains.
Similar to a dense crowd at a concert, if one cell pulls on one side, another cell senses the action and can react by either going in the same direction or pulling the opposite way. Information can then propagate and travel in waves—waves that are visible under a microscope.
“Cells not only sense mechanical forces but also their chemical environment—forces and biochemical signals cells are exerting on each other,” Hannezo continues. “Their communication is an interplay of biochemical activity, physical behavior, and motion; however, the extent of each mode of communication and how such mechanochemical interplays function in living tissues has been elusive until now.”
Predicting movement patterns
Driven by the wave visuals, the scientists' goal was to establish a theoretical follow-up model that would validate their previous theory on how cells move from one region to the next. Daniel Boocock explains, “In our earlier work, we wanted to uncover the biophysical origin of the waves and whether they play a role in organizing collective cell migration. However, we hadn't considered the liquid-solid transition of the tissue, the noise inherent in the system, or the detailed structure of the waves in 2D.”
Their latest computer model pays attention to cell motility and material properties of the tissue. With it, Boocock and Hannezo found how cells communicate mechanically and chemically and how they move. They were able to replicate the phenomena observed in Petri dishes, verifying a theoretical explanation of cell communication based on physical laws.
Testing the theory
For experimental proof, Boocock and Hannezo collaborated with biophysicist Tsuyoshi Hirashima. To rigorously test whether the new model is applicable to real biological systems, scientists used 2D monolayers of MDCK cells—specific mammalian kidney cells—that are a classical in vitro-model for such research. “If we inhibited a chemical signaling pathway that allows cells to sense and generate forces, the cells stopped moving and no communication waves spread,” Hannezo explains. “With our theory, we can easily change different components of the complex system and determine how the dynamics of the tissue adapt.”
What’s next?
Cellular tissue resembles liquid crystals in some ways: it flows like a liquid but is arranged like a crystal. Boocock adds: “In particular, the liquid crystal-like behavior of biological tissue has only been studied independently of mechanochemical waves.” An extension to 3D tissues or monolayers with complex shapes, just as in living organisms, is one possible future avenue of investigation.
The researchers have also begun to optimize the model with regard to wound healing. Where parameters improve the flow of information, healing has been accelerated—in computer simulations. Hannezo adds enthusiastically, “What’s really interesting is how well our model would work for wound healing in cells within living organisms.”